Tyler E. Nordgren
Whether people realize it or not, nearly everyone is familiar with the movement of the night sky. We've all seen the full moon rise in the east in the evening twilight as the sun sets in the west. Towards the north, the constellation of the Big Dipper slowly traces a great circle in the sky, at the center of which is the star Polaris, also known as the North Star. By chance the north pole of our planet points almost directly at Polaris, and so while everything; the Sun, moon, stars and planets arc through the sky as the Earth turns on its axis, Polaris alone is motionless; a constant. Find it in the sky and you will always know which way is north. For such a unique constant, it may be a bit surprising then to realize that Polaris is a variable star. On Anderson Mesa, south of Flagstaff, a new generation of telescope has turned its attention to this variable star in a constant position. Many stars in the sky change brightness over periods ranging from a few days to over a year. If you know where to look and have the patience, some of these variable stars can double in brightness after only a few weeks. While Polaris is not one of these, in fact the amount by which it brightens is quite small, it is still in a very special class of variable stars known as cepheids. In the early 1900's, Harvard College Observatory's Henrietta Leavitt discovered that for variable stars of this type, the more luminous the star (i.e., the brighter it would look if all stars were the same distance away from us) the longer the period of its brightness change. This realization is one of the most important in mapping the universe. If we can measure how long it takes a cepheid in another galaxy to vary its brightness we can learn how intrinsically bright it is. Once we know that we can tell how far away the galaxy must be in order for that star to look as faint as it does from the Earth.
But cepheids can tell us about more than just the locations of stars, they can also tell us about the interiors of stars. To learn about stellar interiors we must first observe the size of their exterior and to do this the newest technology in telescope design is brought to bear on Anderson Mesa, Lowell Observatory's dark sky site. The Navy Precision Optical Interferometer, a new type of telescope being built by the U.S. Naval Observatory with the cooperation of Lowell Observatory has just recently completed observations of Polaris and several other cepheid variables. This new telescope, the NPOI for short, combines the light from three widely spaced telescopes (and some day more) to act as one giant telescope with a diameter as large as the maximum distance between them. The result is that the NPOI can detect and measure the diameters of hundreds of stars in the night sky. For comparison, the Hubble Space Telescope can do this for only one star, Betelgeuse, the star with the largest angular diameter in the night sky.
Until a few years ago, when telescopes like the NPOI came on the scene, the radius of a cepheid was found through only indirect or theoretical means. It was found that like the relation between period and luminosity, there was also a relation between radius and period with bigger cepheids having longer periods. With the new NPOI observations, however, we have finally been able to compare direct observations with these older, indirect, or theoretical ones. For Polaris, the result was surprising.
The reason cepheids get brighter and dimmer is because they are constantly changing size. For this type of star a complex series of events in its core causes the material of which it is made to alternately expand outward and then contract in a perpetual periodic way. For most cepheids, at any given time, all the material making up the star is moving in the same direction; this type of pulsation is called fundamental mode pulsation and is shown in Figure 1 below.

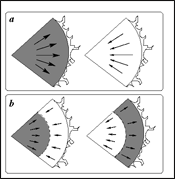 |
Figure 1.
Fundamental and first overtone pulsation. |
For a few cepheids the internal dance of gases is more complicated. At a particular depth in the atmosphere there is a boundary, called a node, inside of which all the gas is moving in one direction while outside the node all the gas is moving in the opposite direction. The pulsation happens as first all the material deeper than the node moves outward, while the material higher than the node moves inward. Once the material deeper than the node stops expanding and falls inward, the gas higher than the node stops falling inward and instead moves out. This type of pulsation is called first overtone pulsation and is shown in Figure 1b. In the event that there are two nodes inside a star, it becomes second overtone pulsation and so on with increasing numbers of nodes. From the outside of the star, however, one kind of pulsation looks just like the other; the star gets bigger, then the star gets smaller. However, since the physics is different between these two kinds of pulsation, there is a difference between the period-radius and period-luminosity relations for fundamental and overtone pulsators, and from this difference we can determine which type of pulsation is at work. The NPOI observations for Polaris show that the North Star has a radius 46 times that of our own Sun. Imagine a solar system like ours around Polaris. A planet at the same distance as the Earth would see a sun the size of a beach ball held at arm's length. With an observed period of 4.0 days, the NPOI observation shown in Figure 2 shows Polaris is too big according to the theoretical prediction. However, if Polaris is pulsating in the first overtone mode, rather than its fundamental mode, then we are comparing apples to oranges. For cepheids the first overtone period is equal to 0.71 times the fundamental period. We should therefore plot Polaris not at 4.0 days but instead at a period of 5.6 days. The arrow in Figure 2 shows this change in Polaris' position on the period-radius diagram and the result is a perfect match with what the theory predicts. Only if Polaris is a first overtone pulsator will models of stellar pulsation agree with the NPOI observations.

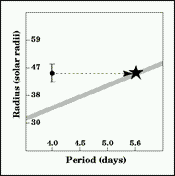 . |
Figure 2.
The period-radius relation for Cephieds |
While stars remain as nothing more than simple points of light in most telescopes (just as they looked through Galileo's first telescope 500 years ago) the NPOI and other telescopes like it are finally resolving them into worlds of their own. And for Polaris, seeing its surface allows us to peer into its depths which brings new light to this variable: our constant guide in the northern sky. |